
Triboelectric Energy Harvesting for Self-powered Antibacterial Applications
This is an Open Access article distributed under the terms of the Creative Commons Attribution Non-Commercial License(https://creativecommons.org/licenses/by-nc/3.0/) which permits unrestricted non-commercial use, distribution, and reproduction in any medium, provided the original work is properly cited.
Abstract
Triboelectric nanogenerators (TENGs) have emerged as a highly promising energy harvesting technology capable of harnessing mechanical energy from various environmental vibrations. Their versatility in material selection and efficient conversion of mechanical energy into electric energy make them particularly attractive. TENGs can serve as a valuable technology for self-powered sensor operation in preparation for the IoT era. Additionally, they demonstrate potential for diverse applications, including energy sources for implanted medical devices (IMDs), neural therapy, and wound healing. In this review, we summarize the potential use of this universally applicable triboelectric energy harvesting technology in the disinfection and blocking of pathogens. By integrating triboelectric energy harvesting technology into human clothing, masks, and other accessories, we propose the possibility of blocking pathogens, along with technologies for removing airborne or waterborne infectious agents. Through this, we suggest that triboelectric energy harvesting technology could be an efficient alternative to existing pathogen removal technologies in the future.
Keywords:
Triboelectric energy harvesting, Microbial inactivation, Air-purification, Water treatment, Antibacterial property1. INTRODUCTION
With the rapid advancement of wearable smart devices and the emergence of the Internet of Things (IoT) era, power consumption of these devices has significantly increased. This leads to several challenges in relying solely on conventional batteries to sustain prolonged operation times for wearable devices. To overcome these energy limitations, the integration of energy harvesting technology as a power source has become crucial. Energy harvesting technology offers the ability to capture and utilize ambient energy from the surrounding environment [1-4]. By harnessing various forms of energy such as light, heat, vibration, or motion, it enables real-time charging of batteries and extends the overall usage time of wearable devices. In some cases, energy harvesting can even eliminate the need for batteries entirely by providing a sufficient level of power to sustain the device's operation, resulting in self-powered systems.
First reported in 2012, triboelectric energy harvesting operates based on the triboelectric effect generated by the friction between two different materials, coupled with electrostatic induction induced through an external circuit, to convert mechanical energy into electrical energy [5]. The advantage of triboelectric energy harvesting lies in the various material combinations that can facilitate the transfer of charges between them. This allows for a diverse selection of materials for the triboelectric device. Furthermore, the versatility of material choices enables low-cost device fabrication, and unlike other energy harvesting technologies, triboelectric energy harvesting can be easily scaled up for large-scale applications [6].
Triboelectric energy harvesting technology is highly suitable for powering self-sustaining sensors, and substantial research has focused on developing wearable sensors that can be self-powered by utilizing flexible materials or fabric-based approaches. This research demonstrates the active pursuit of harnessing the potential of triboelectric energy harvesting for enabling wearable sensors [7-10]. Furthermore, research focuses on applying triboelectric energy harvesting as an energy source for low-power devices or battery charging. This research involves the development of high-electrical output materials, optimization of TENG structures, and modification of circuit configurations [11-13]. Recently, significant research investigated the potential of triboelectric energy harvesting as an energy source for implantable medical devices (IMDs). Studies have explored various approaches, including ultrasound- and inertial-based triboelectric energy harvesting, to effectively charge the batteries within these devices. In addition, ongoing research focuses on harnessing energy generated within the human body for applications such as nerve stimulation and therapy. Furthermore, several research studies have focused on the development of transient electronics using biodegradable materials that dissolve after completing their therapeutic function [14-16].
Recently, the outbreak of COVID-19 pandemic has considerably affected global economic and public health. Consequently, research studies using triboelectric energy harvesting technology to inactivate pathogen has been widely conducted [17]. This review provides a comprehensive overview of the recent advancements in self-powered systems based on TENGs for disinfection applications in various environments. Specifically, we highlight the notable outcomes and practical implementations of TENGs in various antibacterial applications, focusing on two key areas: airborne/waterborne disinfection and human healthcare applications.
2. TRIBOELECTRIC ENERGY HARVESTING FOR MICROBIAL DISINFECTION APPLICATIONS
2.1 Triboelectric energy harvesting for airborne and waterborne disinfection
Fig. 1 presents the potential of triboelectric energy harvesting technology for the removal of pathogens in airborne environments. Fig. 1(a) demonstrates the airborne disinfection system using triboelectric energy harvesting technology to inactivate the pathogens floating in the air. The disinfection system operates as follows: the triboelectric charges generated by the TENG is applied to the (-) electrode and the (+) electrode. The (-) electrode induces a negative charge on the pathogen surfaces, leading to their electrostatic attraction on the (+) electrode. Subsequently, Cu3P nanowires on the (+) electrode localize a high electric field at the tip of the nanowires. This localized electric field causes surface damage to the pathogens through electroporation, leading to their inactivation. This approach demonstrated a high efficiency in removing pathogens even at high air-flow rates [18]. Fig. 1(b) shows that the high-performance triboelectric material generates substantial electrical power, resulting in the production of ozone, which effectively eliminates pathogens. However, this method has the drawback of being highly time consuming (40 min) for ozone generation to attain an effective sterilization rate [19]. Fig. 1(a)–(b) describes various approaches for removing airborne pathogens, such as utilizing antimicrobial substances, electroporation-based elimination, and ozone generation. This airborne disinfection technology can be a remarkable advancement from an industrial perspective since there are no external power sources to operate this disinfection system, enabling efficient removal of pathogens in the air.

Triboelectric energy harvesting for airborne disinfection applications. (a) Airborne disinfection with the electroporation of localized electric field driven by TENG. Reprinted with permission from Ref. [18] Copyright (2021) Springer Nature. (b) Airborne disinfection using ozone generated by high-performance TENG. Reprinted with permission from Ref. [19] Copyright (2023) IOP Publishing.
In Fig. 2(a), we focus on disinfecting the pathogens that inhabit water environments. In Fig. 2(a), hand-powered TENG is demonstrated to release copper (Cu), which has antibacterial effect and damages the membrane and infiltrates the cell, inducing an oxidative stress response involving endogenous reactive oxygen species (ROS). The generation of Cu2+ ions from the internal Cu wire of the TENG confirmed the pathogen disinfection resulting from copper ionization. Furthermore, it was proven that the amount of released copper ions was below the maximum contaminant level goal (MCLG) of Cu for drinking water set by the US Environmental Protection Agency [20]. In Fig. 2(b), the high-rotating-speed TENGs is demonstrated using hand-powered (~7,500 rpm), generating high voltage and current, to achieve efficient pathogen removal and water disinfection by employing electroporation and reactive oxygen species (ROS). This technology exhibited a high level of pathogen disinfection efficacy based on high voltage electroporation and water disinfection capabilities [21].

Triboelectric energy harvesting for waterborne disinfection applications. (a) Waterborne disinfection system using antibacterial. Effect of Cu2+ ions. Reprinted with permission from Ref. [20] Copyright (2019) John Wiley and Sons (b) Waterborne disinfection system based on electroporation and ROS through high-speed TENGs. Reprinted with permission from Ref. [21] Copyright (2022) John Wiley and Sons (c) Waterborne disinfection system using electroporation of PEDOT/PPy-Cell TENG. Reprinted with permission form Ref. [22] Copyright (2021) Elsevier.
In Fig. 2(c), a prototype cup was proposed to prove the disinfection of waterborne pathogens through electroporation using TENG. By passing the water through the filtration stages thrice with the aid of TENG, most waterborne pathogens were effectively eliminated [22]. Fig. 2(a)–(c) focus on the disinfection of waterborne pathogens, employing antimicrobial substances and electroporation-based elimination. These methods offer a novel paradigm to enhance public health by effectively eliminating waterborne pathogens, particularly in third-world countries with inadequate water disinfection capabilities.
2.2 Triboelectric energy harvesting for human healthcare
In Fig. 3, we introduce the triboelectric energy harvesting technologies for pathogen blocking and removal to enhance human healthcare. In Fig. 3(a), the research study is presented that utilizes human biomechanical energy to continuously apply a negative charge (-) to masks, effectively blocking pathogens. The study specifically validated the blocking efficiency of masks using the prevalent SARS-CoV-2 virus at that time, and it measured the charge on the surface of the virus, confirming its negative charge [23]. In Fig. 3(b), the fabrication of a nanofiber membrane (NFM) using electrospinning is demonstrated, incorporating antibacterial properties into a polybutylene adipate terephthalate (PBAT) matrix. By enhancing the friction characteristics, this approach not only increased the blocking efficiency for pathogens but also for fine particles [24]. Fig. 3(c) utilized textiles suitable for real-life applications to analyze their frictional electrical properties. By employing a fabric structure capable of exhibiting a large frictional surface area, the verification of pathogen blocking was conducted. Moreover, this paper proposed the direct application of triboelectric charges, using the existing TENG technology, for pathogen blocking instead of relying on traditional TENG-based pathogen-blocking methods. This suggests the potential for immediate real-life implementation [25]. Fig. 3(a)–(c) provide evidence for efficient pathogen blocking using fabrics that can be used for mask and clothing production, employing frictional electricity. In Fig. 4(a), we focus on pathogen removal in patch-type devices that can be attached to the human body. In Fig. 4(a), a wound healing patch is shown, which was developed using recombinant spider silk proteins (RSSP). To prevent infection at the wound site, pathogens were eliminated using the electric field of a TENG and converted into reactive oxygen species (ROS) [26]. In Fig. 4(b), a breathable, biodegradable, and antibacterial e-skin based on all-nanofiber TENGs was created. In this TENG, silver nanowires (AgNWs) were placed between layers of polylactic-co-glycolic acid (PLGA) and polyvinyl alcohol (PVA) to allow the release of silver ions, thus providing antibacterial properties through a porous structure [27]. Fig. 4(c) focused on preventing surgical site infections (SSI) by producing a device using biodegradable materials, which was inserted into the surgical site. To eliminate infectious pathogens, high frequency with no effect on human body such as ultrasound was proposed. The mechanism involved the induction of charges on the pathogen's surface due to the polarization caused by ultrasound, leading to surface damage and subsequent self-elimination of the pathogens. This approach effectively removed potentially infectious pathogens within the surgical site [28]. Fig. 4(a)–(c) provide a concise overview of research related to pathogen removal in patch-type devices that can be attached to the human body, as well as efforts to prevent infection at surgical sites.
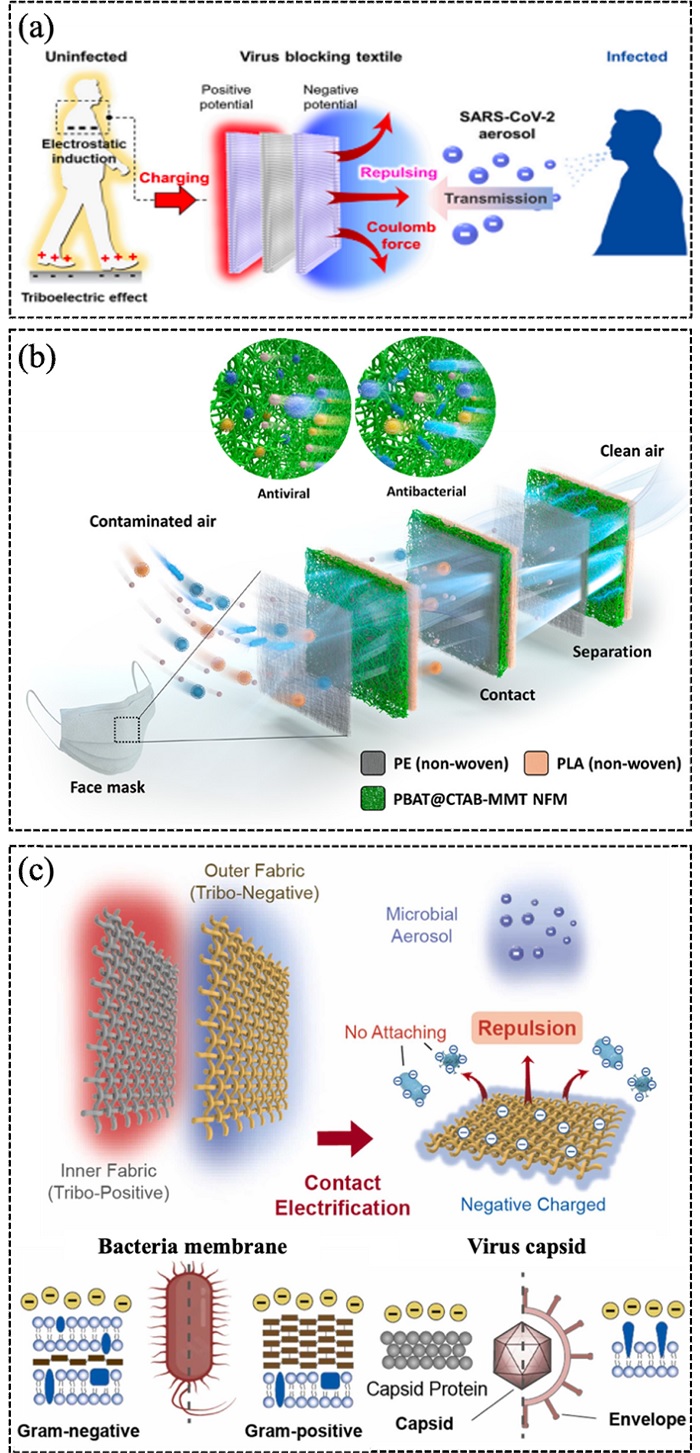
Triboelectric energy harvesting for wearable mask/clothes healthcare applications. (a) Virus blocking textiles powered by a human body’s triboelectric energy harvesting. Reprinted with permission from Ref. [23] Copyright (2022) Cell Press. (b) Pathogens trapped by nanofiber membrane using electrospinning. Reprinted with permission from Ref. [24]. Copyright (2022) American Chemical Society. (c) Triboelectric charge-induced electrostatic repulsion between fabric and microbial aerosol. Reprinted with permission from Ref. [25] Copyright (2023) Elsevier.

Triboelectric energy harvesting for patchable healthcare applications. (a). Recombinant spider silk proteins induced ROS through the TENG. Reprinted with permission from Ref. [26] Copyright (2018) John Wiley and Sons. (b). Antibacterial effect of silver ions released by AgNWs. Reprinted with permission from Ref. [27] Copyright (2020) American Association for the Advancement of Science. (c) Ultrasounddriven on-demand TENG to prevent surgical site infections. Reprinted with permission from Ref. [28] Copyright (2023) John Wiley and Sons.
3. CONCLUSION
Triboelectric energy harvesting technology possesses advantages such as the versatility of material selection, low-cost production feasibility, scalability, and high energy conversion efficiency for converting various mechanical vibrational energies, ranging from low to high frequencies, into electrical energy. It shows potential for self-powered sensor operation and can be used as energy sources for low-power electronic devices, as blue energy in diverse environments such as vibrations and wind in industrial settings or wave motion in the ocean, along with applications in areas such as implanted medical devices, neural therapy, and wound healing. In this review, we aim to summarize the content related to pathogen removal and blocking, which is another research area relevant to public health. By utilizing the characteristic high electric field generated by TENG, various studies have focused on removing airborne pathogens or eliminating waterborne pathogens to enhance public health. Additionally, we introduce studies on blocking and removing pathogens in applications such as masks, textiles, and patch-type devices attached to the human body, thereby demonstrating another promising application area for the TENG technology. However, despite the advancements in pathogen blocking and removal technology based on triboelectric energy harvesting, some challenges still need to be addressed. In waterborne pathogen inactivation, the time required for disinfection is still lengthy, and a separate sterilization process is necessary as the current focus remains on pathogen deactivation. Increasing the speed of water treatment along with high-efficiency pathogen removal can be a way to address this problem. In airborne pathogen removal and blocking technology, there is a need to verify the blocking efficiency in high-humidity or high-temperature environments that can decrease the triboelectric energy harvesting. Once these issues are addressed, the triboelectric energy harvesting-based pathogen removal and blocking technology is expected to have high commercialization potential in the future.
Acknowledgments
The authors acknowledge financial support by the Basic Science Research Program (2022R1A3B1078291, Research Leader Program) through the National Research Foundation of Korea (NRF) grants funded by the Korean government (MSIT). S.-W.K. acknowledges the Yonsei World-Class Fellow Program funded by Youn Jae Lee.
REFERENCES
-
Z. L. Wang and J. Song, “Piezoelectric Nanogenerators Based on Zinc Oxide Nanowire Arrays”, Science, Vol. 312, No. 5771, pp. 242-246, 2006.
[https://doi.org/10.1126/science.1124005]
-
X. Wang, J. Song, J. Liu, and Z. L. Wang, “Direct-Current Nanogenerator Driven by Ultrasonic Waves”, Science, Vol. 316, No.5821, pp. 102-105, 2007.
[https://doi.org/10.1126/science.1139366]
-
Y. Hu and Z. L. Wang, “Recent progress in piezoelectric nanogenerators as a sustainable power source in self-powered systems and active sensors”, Nano Energy, Vol. 14, pp. 3-14, 2015.
[https://doi.org/10.1016/j.nanoen.2014.11.038]
-
S. Cju, Y. Cui, and N. Liu, “The path towards sustainable energy”, Nat. Mater., Vol 16, No. 1, pp. 16-22, 2017.
[https://doi.org/10.1038/nmat4834]
-
F. R. Fan, Z. Q. Tian, and Z. L. Wang, “Flexible triboelectric generator”, Nano Energy, Vol. 1, No. 2, pp. 328-334, 2012.
[https://doi.org/10.1016/j.nanoen.2012.01.004]
-
S. S. Kwak, H. J. Yoon, and S. W. Kim, “Textile-Based Triboelectric Nanogenerators for Self-Powered Wearable Electronics”, Adv. Funct. Mater., Vol. 29, No. 2, p. 1804533, 2019.
[https://doi.org/10.1002/adfm.201804533]
-
Z. Zhao, C. Yan, Z. Liu, X. Fu, L. M. Peng, Y. Hu, and Z. Zheng, “Machine-Washable Textile Triboelectric Nanogenerators for Effective Human Respiratory Monitoring through Loom Weaving of Metallic Yarns”, Adv. Mater., Vol. 28, No. 46, pp. 10267-10274, 2016.
[https://doi.org/10.1002/adma.201603679]
-
X. Pu, W. Song, M. Liu, C. Sun, C. Du, C. Jiang, X. Huang, D. Zou, W. Hu, and Z. L. Wang, “Wearable Power-Textiles by Integrating Fabric Triboelectric Nanogenerators and Fiber-Shaped Dye-Sensitized Solar Cells”, Adv. Energy Mater., Vol. 6, No. 20, p. 1601048, 2016.
[https://doi.org/10.1002/aenm.201601048]
-
F. Yi, L. Lin, S. Niu, P. K. Yang, Z. Wang, J. Chen, Y. Zhou, Y. Zi, J. Wang, Q. Liao, Y. Zhang, and Z. L. Wang, “Stretchable-Rubber-Based Triboelectric Nanogenerator and Its Application as Self-Powered Body Motion Sensors”, Adv. Funct. Mater., Vol. 25, No. 24, pp. 3688-3696, 2015.
[https://doi.org/10.1002/adfm.201500428]
-
P. Bai, G. Zhu, Q. Jing, J. Yang, J. Chen, Y. Su, J. Ma, G. Zhang, and Z. L. Wang, “Membrane-Based Self-Powered Triboelectric Sensors for Pressure Change Detection and Its Uses in Security Surveillance and Healthcare Monitoring”, Adv. Funct. Mater., Vol. 24, No. 37, pp. 5807-5813, 2014.
[https://doi.org/10.1002/adfm.201401267]
-
R. Zhang and H. Olin, “Material choices for triboelectric nanogenerators: A critical review”, EcoMat, Vol. 2, No. 4, p. e12062, 2020.
[https://doi.org/10.1002/eom2.12062]
-
Y. Zhu, T. Zhao, F. Sun, C. Jia, H. Ye, Y. Jiang, K. Wang, C. Huang, Y. Xie, and Y. Mao, “Multi-functional triboelectric nanogenerators on printed circuit board for metaverse sport interactive system”, Nano Energy, Vol. 113, p. 108520, 2023.
[https://doi.org/10.1016/j.nanoen.2023.108520]
-
H. Xiang, Y. Zeng, X. Huang, N. Wang, X. Cao, and Z. L. Wang, “From Triboelectric Nanogenerator to Multifunctional Triboelectric Sensors: A Chemical Perspective toward the Interface Optimization and Device Integration”, Small, Vol. 18, No. 43, p. 210722, 2022.
[https://doi.org/10.1002/smll.202107222]
-
R. Hinchet, H. J. Yoon, H. Ryu, M. K. Kim, E. K. Choi, D. S. Kim, and S. W. Kim, “Transcutaneous ultrasound energy harvesting using capacitive triboelectric technology”, Science, Vol. 365, No. 6452, pp. 491-494, 2019.
[https://doi.org/10.1126/science.aan3997]
-
H. Ryu, H. Park, M. K. Kim, B. Kim, H. S. Myoung, T. Y. Kim, H. J. Yoon, S. S. Kwak, J. Kim, T. H. Hwang, E. K. Choi, and S. W. Kim, " Self-rechargeable cardiac pacemaker system with triboelectric nanogenerators”, Nat. Commun., Vol. 12, No. 1, pp. 4374(1)-4374(9), 2021.
[https://doi.org/10.1038/s41467-021-24417-w]
-
D. M. Lee, N. Rubab, I. Hyun, W. Kang, Y. J. Kim, M. Kang, B. O. Choi, and S. W. Kim, “Ultrasound-mediated triboelectric nanogenerator for powering on-demand transient electronics”, Sci. Adv., Vol. 8, No. 1, p. eabl8423, 2022.
[https://doi.org/10.1126/sciadv.abl8423]
-
G. Q. Gu, C. B. Han, J. J. Tian, T. Jiang, C. He, C. X. Lu, Y. Bai, J. H. Nie, Z. Li, and Z. L. Wang, “Triboelectric nanogenerator enhanced multilayered antibacterial nanofiber air filters for efficient removal of ultrafine particulate matter”, Nano Res., Vol. 11, No. 8, pp. 4090-4101, 2018.
[https://doi.org/10.1007/s12274-018-1992-1]
-
Z. Y. Huo, Y. J. Kim, I. Y. Suh, D. M. Lee, J. H. Lee, Y. Du, S. Wang, H. J. Yoon, and S. W. Kim, “Triboelectrification induced self-powered microbial disinfection using nanowire-enhanced localized electric field”, Nat. Commun., Vol. 12, No. 1, pp. 3693(1)-3693(11), 2021.
[https://doi.org/10.1038/s41467-021-24028-5]
-
J. He, X. Guo, C. Pan, G. Cheng, M. Zheng, Y. Zi, H. Cui, and X. Li, “High-output soft-contact fiber-structure triboelectric nanogenerator and its sterilization application”, Nanotechnol., Vol. 34, No. 38, p. 385403, 2023.
[https://doi.org/10.1088/1361-6528/acdfd5]
-
W. Ding, J. Zhou, J. Cheng, Z. Wang, H. Guo, C. Wu, S. Xu, Z. Wu, X. Xie, and Z. L. Wang, “TriboPump: A Low-Cost, Hand-Powered Water Disinfection System”, Adv. Energy Mater., Vol. 9, No. 27, p. 1901320, 2019.
[https://doi.org/10.1002/aenm.201901320]
-
Z. Y. Huo, D. M. Lee, J. M. Jeong, Y. J. Kim, J. Kim, I. Y. Suh, P. Xiong, and S. W. Kim, “Microbial Disinfection with Supercoiling Capacitive Triboelectric Nanogenerator”, Adv. Energy Mater., Vol. 12, No. 15, p. 2103680, 2022.
[https://doi.org/10.1002/aenm.202103680]
-
S. Cho, Z. Hanif, Y. Yun, Z.A. Khan, S. Jang, Y. Ra, Z. H. Lin, M. La, S. K. Park, and D. Choi, “Triboelectrification-driven microbial inactivation in a conductive cellulose filter for affordable, portable, and efficient water sterilization”, Nano Energy, Vol. 88, p. 106228, 2021.
[https://doi.org/10.1016/j.nanoen.2021.106228]
-
M. Kang, N. Y. Jang, Y. J. Kim, H. J. Ro, D. Kim, Y. Kim, H. T. Kim, H. M. Kwon, J. H. Ahn, B. O. Choi, N. H. Cho, and S. W. Kim, “Virus blocking textile for SARS-CoV-2 using human body triboelectric energy harvesting”, Cell Rep. Phys. Sci., Vol. 3, No. 4, pp. 100813(1)-100813(12), 2022.
[https://doi.org/10.1016/j.xcrp.2022.100813]
-
Y. Cho, Y. Son, J. Ahn, H. Lim, S. Ahn, J. Lee, P. K. Bae, and I. D. Kim, “Multifunctional Filter Membranes Based on Self-Assembled Core–Shell Biodegradable Nanofibers for Persistent Electrostatic Filtration through the Triboelectric Effect”, ACS Nano, Vol. 16, No. 11, pp. 19451-19463, 2022.
[https://doi.org/10.1021/acsnano.2c09165]
-
I. Y. Suh, Y. J. Kim, P. Zhao, D. S. Cho, M. Kang, Z. Y. Huo, and S. W. Kim, “Self-powered microbial blocking textile driven by triboelectric charges”, Nano Energy, Vol. 110, p. 108343, 2023.
[https://doi.org/10.1016/j.nanoen.2023.108343]
-
Y. Zhang, Z. Zhou, L. Sun, Z. Liu, Z. Zia, and T. H. Tao, “Genetically Engineered” Biofunctional Triboelectric Nanogenerators Using Recombinant Spider Silk”, Adv. Mater., Vol. 30, No. 50, p. 1805722, 2018.
[https://doi.org/10.1002/adma.201805722]
-
X. Peng, K. Dong, C. Ye, Y. Jiang, S. Zhai, R. Cheng, D. Liu, X. Gao, J. Wang, and Z. L. Wang, “A breathable, biodegradable, antibacterial, and self-powered electronic skin based on all-nanofiber triboelectric nanogenerators”, Sci. Adv., Vol. 6, No. 26, pp. eaba9624(1)-eaba9624(10), 2020.
[https://doi.org/10.1126/sciadv.aba9624]
-
I. M. Imani, B. Kim, X. Xiao, N. Rubab, B. J. Park, Y. J. Kim, P. Zhao, M. Kang, and S. W. Kim, “Ultrasound-Driven On-Demand Transient Triboelectric Nanogenerator for Subcutaneous Antibacterial Activity”, Adv. Sci., Vol. 10, No. 3, pp. 2204801(1)-2204801(9), 2023.
[https://doi.org/10.1002/advs.202204801]