
Development of Stretchable Electronics Using Geometric Strategies and Applications
This is an Open Access article distributed under the terms of the Creative Commons Attribution Non-Commercial License(https://creativecommons.org/licenses/by-nc/3.0/) which permits unrestricted non-commercial use, distribution, and reproduction in any medium, provided the original work is properly cited.
Abstract
Soft and stretchable electronics, equipped with diverse functional devices, have recently garnered attention owing to their versatility in applications such as stretchable displays, flexible batteries, and electronic skin (e-skin). A fundamental challenge in realizing stretchable electronics lies in conferring the necessary flexibility to crucial electrical components such as electrodes and devices. However, the prevalent electronic materials, exhibit limited stretchability, presenting a significant obstacle to the advancement of soft and stretchable electronics. To overcome this challenge, various strategies rooted in geometrical engineering have been explored to enhance the adaptability of rigid materials. This study delves into the realm of geometrical engineering by, examining techniques such as serpentine patterns, kirigami-inspired designs, and island structures, with a keen focus on recent progress and future prospects.
Keywords:
Stretchable electronics, Geometrical engineering, Serpentines, Kirigami, E-skin1. INTRODUCTION
Stretchable electronics are electronic devices with high flexibility and deformability, unlike conventional electronics with fixed shapes [1,2]. Stretchable electronics maintain their functionality under manual stretching or bending, making them suitable for attaching to curved surfaces of the human body without deformation. Owing to these outstanding characteristics, stretchable electronics can be applied to various stretchable electronic components, in addition to stretchable displays [3], stretchable batteries, and wearable electronic skin for health monitoring, by integrating chips and sensors with various functionalities.
To develop stretchable electronics, materials with low strain (<3%) are required for electrodes, substrates, and components (sensors and, chips) to impart flexibility. Consequently, research on geometric designs and manufacturing strategies applicable to commonly used substrates, such as polymer films, metal films, and rigid inorganic materials, is being actively conducted globally. In particular, geometric designs based on finite element analysis (FEA) have demonstrated the feasibility of stretchable electronics, including serpentine structures [4-7], kirigami [8], and island structures [9-12].
In this paper, we introduce materials and components based on geometric design structures, including serpentine, kirigami, and island structures. Moreover, we present various types of stretchable displays, stretchable batteries, heating elements, and electronic skins implemented using these designs (Fig. 1).
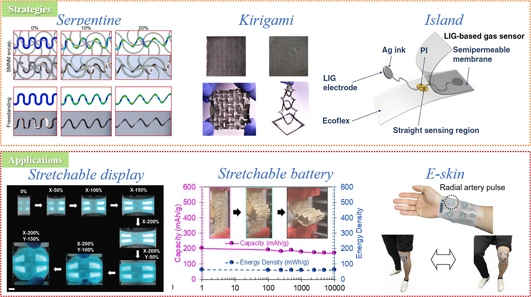
Serpentine “Reprinted with permission from Ref. [13]. Copyright (2022) American Association for the Advancement of Science”, kirigami “Reprinted with permission from Ref. [14]. Copyright (2022) American Chemical Society”, and island structures “Reprinted with permission from Ref. [15]. Copyright (2022) Springer Nature” that enable stretchable electronics. Various application of stretchable displays “Reprinted with permission from Ref. [16]. Copyright (2022) Wiley-VCH GmbH”, stretchable batteries “Reprinted with permission from Ref. [17]. Copyright (2023) The Royal Society of Chemistry”, and electronic skin (e-skin) “Reprinted with permission from Ref. [18]. Copyright (2022) American Chemical Society” implemented by applying these geometrical designs.
2. The Trends in Geometric Structural Strategies for Enabling Stretchable Electronics
Many scholars have been working toward imparting flexibility to the entire electronic system to enable the integration of flexible and stretchable electronic components into wearable devices. In particular, various geometric structures play a pivotal role in enhancing the tensile strain in the horizontal direction. In this context, we introduce the principles of geometric structures and present research cases in which these structures have significantly improved the maximum strain tolerance of electronic components.
2.1 Trends in Serpentine Structure Strategy
A serpentine electrode is a structure designed with a winding and snake-like pattern deposited on a rigid substrate with a metal layer. Serpentine structures feature convoluted, snake-like patterns instead of sharp corners to enable free geometric movements. The rationale behind this design starts by considering the curved shape of the serpentine to be analogous to a one-dimensional spring. Owing to their self-similar characteristics, the repeating curves exhibit spring-like behavior. This motion occurs in both vertical (y-axis) and horizontal (x-axis) directions, making it a systematically repeatable “foldable” design structure. Expanding these serpentine patterns sideways results in stretching, while introducing additional geometric transformations in the vertical direction of the plane. Consequently, this design effectively distributes the concentrated stress, preventing stress on the metal film, which is susceptible to strain, thereby imparting flexibility. Serpentine structures can have a coefficient similar to that of the human skin (~100 kPa), making them suitable for attachment to human skin. However, excessive stretching can lead to irreversible deformation; therefore, an appropriate structure with a strain of 20–50% is required.
John A. Rogers et al. designed serpentine structures using a finite element method (FEM) based on polyimide materials that can stretch up to 80% [19]. Furthermore, the proposed serpentine structures could withstand up to 140% strain in a 2-dimensional (2 × 2) array configuration (Fig. 2(a),2(b)). Sabrina M. Curtis et al. developed freestanding thin-film TiNiCuCo super elastic alloys for stretchable interconnects [20]. The serpentine-shaped stretchable interconnects limited the local strain and achieved a maximum strain of 153%. The electrical resistivity of the developed electrode in the austenite phase was 5.44 × 10-7 Ω·m, which is higher than that of copper films (1.88 × 10-8 Ω·m) (Fig. 2(c) to 2(d)).
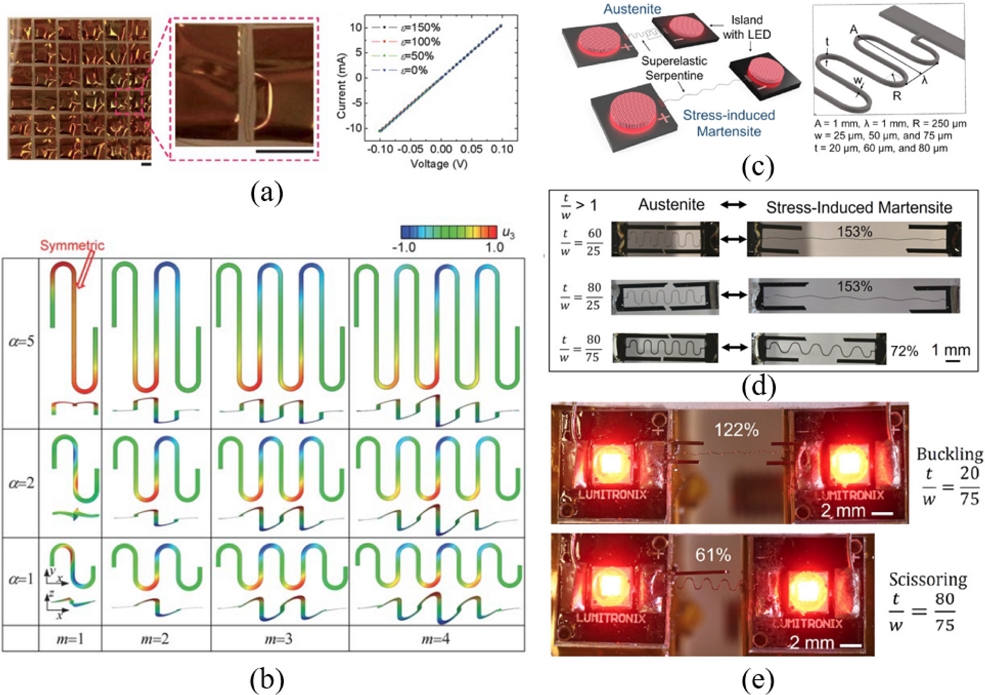
Serpentine structure for stretchable electronics. (a) Optical images displaying a 6 × 6 array of island bridge structures. I–V curves illustrating the performance of serpentine interconnects under varying levels of biaxial strain (0, 50, 100, and 150%). Reprinted with permission from Ref. [19]. Copyright (2013) The Royal Society of Chemistry. (b) Analysis of out-of-plane displacement distribution for buckling modes of serpentine interconnects. (c) A 3D schematic depicts the "island plus serpentine" stretchable design, featuring a superelastic serpentine interconnect in equilibrium within the austenite phase and when stretched under stress-induced martensite conditions. Reprinted with permission from Ref. [20]. Copyright (2023) Springer Nature. (d) Illustration of scissoring deformation (t/w > 1) for serpentine with various t/w ratios, including 60/25, 80/25, and 80/75. (e) Illustration of Cu/TiNiCuCo/Cu serpentine, combining the benefits of superelastic films with the electrical advantages of Cu thin films.
2.2. Trends in Kirigami Structure Strategy
Kirigami is a patterning technique inspired by three-dimensional structures created by drawing repetitive lines on a flat sheet of paper, followed by cutting with a knife and pulling the paper. Kirigami structures involve pattern cutting, which causes the cutting paths to protrude when the device is folded or unfolded. The transformations induced by the kirigami patterns result in small deformations within the plane because each pattern causes localized deformation, whereas the edges extend outward when subjected to tension. Further expansion leads to kirigami patterns that deforming outward from the plane. Finally, the pattern spacing narrowed when the device was folded back into a flat state. Kirigami patterning minimizes the inherent deformations of the substrate, enabling us to artificially impart strain to materials that are typically non-deformable.
Recently, Ahn et al. created a stretchable strain sensor using kirigami-cut polyvinylidene fluoride (PVDF) films [21]. This PVDF film was validated for biocompatibility and achieved 400 % stretchability through structural distortion of the cut parts (Fig. 3(a)-3(c)). Choi et al. implemented a LIB based on auxetic-cut kirigami patterning [22]. They adjusted the segmentation hierarchy and number of segmentations to minimize the deformation applied to the substrate. LIB exhibited 100% stretchability, maintaining stable current density and areal discharged capability even after 70 cycles of strain deformation (Fig. 3(d),3(e)).
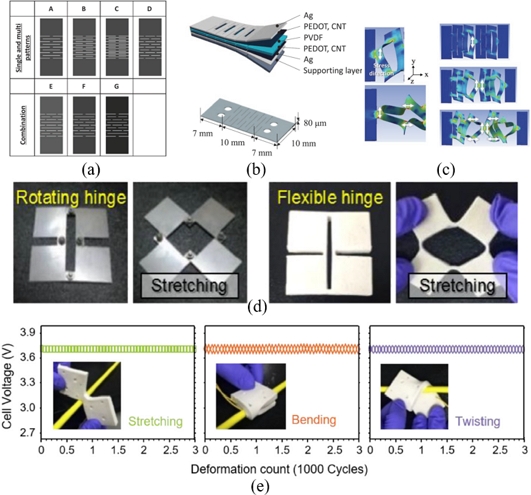
Kirigami structures for stretchable electronics. (a) Design of single, multiple, and combined kirigami patterns. Reprinted with permission from Ref. [21]. Copyright (2023) Wiley-VCH GmbH. (b) Final dimensions of the PVDF strain sensor with kirigami cuts. (c) FEA results for the single and combination patterns. (d) Mechanical performance and output voltage of the auxetic-cut representative volume element (RVE). Reprinted with permission from Ref. [22]. Copyright (2019) Wiley-VCH GmbH. (e) Steady output voltage performance of a dual-unit lithium-ion battery (LIB) subjected to cyclic deformation involving stretching, bending, and twisting.
2.3. Trends in Island Structure Strategy
Research on island structures is actively underway to address the vulnerability to lateral deformations in stretchable electronics. The approach involves placing functional components on rigid islands and encapsulating them with polymers having a lower relative Young's modulus than the islands, thereby minimizing the strain applied to the components.
Several approaches have been reported for enhancing the interfacial stability between islands and polymers. Zhang et al. presented research results that integrated theoretical analysis, numerical simulations, and experiments on the widely used “island effect” in stretchable inorganic electronics [23]. To achieve better performance, they developed buffer layers to enhance the flexibility of the deformable interconnects. The strategy was experimentally proven to be applicable to both two-dimensional (2D) serpentine and three-dimensional (3D) helical interconnects. In the serpentine structures, a simple buffer layer exhibited 1.5 times the performance, whereas a ring buffer layer demonstrated twice the performance (Fig. 4(a) to 4(c)). Yu et al. fabricated slim silicon nanowire (SiNW) arrays with diameters less than 80 nm using an in-plane solid-liquid-solid (IPSLS) mechanism [24]. The SiNW FETs they fabricated exhibited up to 50% strain and maintained their performance even after 1000 cycles (under 20% strain). Furthermore, they operated reliably for 270 days without passivation protection. This system is applicable to flexible displays and wearable electronics (Fig. 4(d),4(e)). The research results of the developed island structures confirmed their potential for stretchable electronics. However, the interface between the islands and polymers is vulnerable to high strain and repeated stretching, and defects at the interface can ultimately lead to component malfunctions. Therefore, further research is required to reduce the size of these islands and produce highly integrated stretchable electronics.
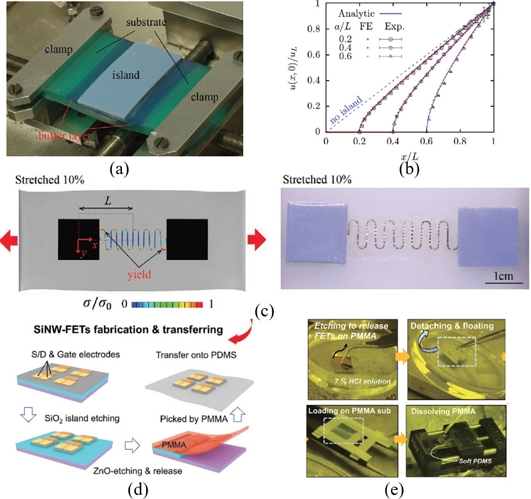
Island structure for stretchable electronics. (a) Illustration of the buffer layer approach for mitigating the island effect. Reprinted with permission from Ref. [23]. Copyright (2022) Wiley-VCH GmbH. Experimental setup designed for the tensile test involving a buffer layer. (b) Influence of island size a/L on u(x, 0), characterized by fitting parameters N = 0.294, 0.427, and 0.494 for a/L values of 0.2, 0.4, and 0.6, respectively. (c) FEA and experimental results for the stretched configuration illustrating the normalized Mises stress distribution within the serpentine interconnects. (d) Description of the fabrication steps for SiNW FET carried out on a stretchable PDMS thin film substrate. Reprinted with permission from Ref. [24]. Copyright (2022) Wiley-VCH GmbH. (e) The specific fabrication process for the SiNW-FETs was subsequently applied to a soft PDMS thin film via batch transfer.
3. Trends in Geometric Structure-Based Stretchable Displays, Batteries, Medical Devices, and Electronic Skin Technologies
Research on stretchable electronic components has enabled diverse applications in the field of electronic skins by combining various functional chips and components.
Choi et al. presented a stretchable battery with a kirigami structure, including a LIB [22]. They confirmed that the battery performance remained intact even after multiple folds and stretches (Fig. 5(a),5(b)). Jacobs used photolithography to create a highly stretchable display based on serpentine electrodes, demonstrating a maximum strain of 260% (Fig. 5(c)-5(e)) [25]. Moreover, they implemented an active matrix within Ecoflex using vertical interconnect access (VIA) technology. Cu, a highly conductive metal, was deposited on the serpentine structure for interconnections.
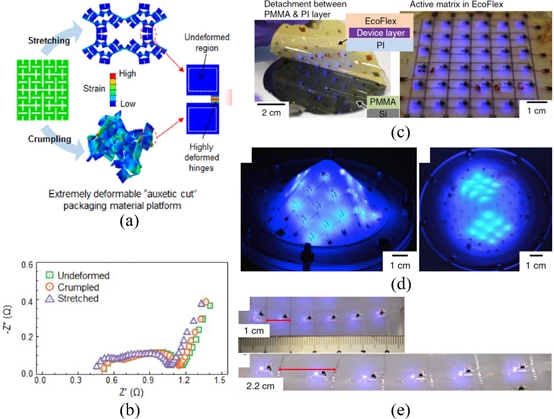
Applications of stretchable batteries and stretchable displays. (a) Design concept for a shape-reconfigurable battery system that employs auxetic cut patterning accompanied by a schematic representation of a flexible battery structure created using auxetic cutting techniques [22]. (b) AC impedance spectra analysis of the auxetic-cut battery under various conditions, including the battery in its intact form when stretched and crumpled. (c) Evaluation and functionality testing of the final device on EcoFlex substrate. Reprinted with permission from Ref. [25]. Copyright (2019) Springer Nature. The device was embedded in a rubber matrix and displayed both a transistor and a light-emitting diode (LED). (d) Achieving a deformable LED active matrix via the stretchable printed circuit boar (SPCB) method. (e) Presentation of stretching test results using a stress-adaptive meander-shaped metal track. The tests were conducted under the unstretched condition and while elongated to 220%.
Kong et al. utilized an LA/DOX composite and thermoplastic polyurethane (TPU) to create an electronic patch for wearable cancer treatment [26]. They employed soft composite nanofibers and compliant electrically resistive heaters. This patch adhered securely to the surgical wounds and exhibited a maximum strain performance of approximately 500–600%. In addition, they developed a wearable system that could be connected to smartphones, enabling remote treatment commands (Fig. 6(a)-6(d)). Ren et al. developed a recyclable electro-thermal electronics system using cellulose and oxidation-resistant copper, offering flexibility and stretchability in a multilayer configuration [27]. The thermal performance of conformal Cu paper can be modulated. The flexible nanocellulose-based material possesses a low thermal conductivity of 15 mW/mK and high thermal resistance below 250 °C. The printed copper traces provide excellent electromagnetic shielding, resulting in a low operating voltage ranging from 0.15 V (37.2 °C) to 0.35 V (88.7 °C) (Fig. 6(e),6(f))
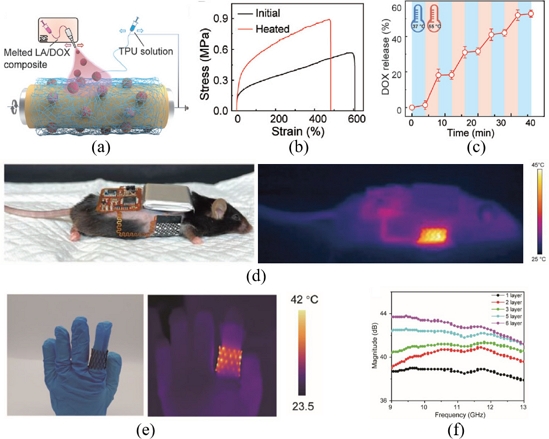
Transparent kirigami heater and its electrothermal performance. (a) Fabrication of a compliant composite nanotextile dressing: Schematic depiction of the co-assembly process employed to produce the composite nanofiber textile. Reprinted with permission from Ref. [26]. Copyright (2023) Wiley-VCH GmbH. (b) Mechanical characterization of the composite nanotextile: Uniaxial stress-strain curves that illustrate the behavior of the composite nanotextile in its pristine state and after undergoing thermal treatment. (c) Controlled drug release from composite nanotextiles: Examination of the release profile under thermal actuation cycles. (d) Integration of an electronic system on a moving subject: Optical image and corresponding infrared camera image showing a fully integrated electronic system operating on a freely moving mouse. (e) Kirigami-inspired wearable heater demonstrates effective heating on a finger using a low driving voltage of 0.15 V. Reprinted with permission from Ref. [27]. Copyright (2023) Elsevier Ltd. (f) Variation in electromagnetic interference (EMI) shielding effectiveness (SE) observed with bending cycles, particularly at a 120° bending angle.
E-skin technology, which resembles the human skin, is crucial for various applications in the field of medical devices, enabling improved disease diagnosis, physiological health monitoring, and the development of interfaces for prosthetics, computer systems, wearable robot devices, and control interfaces. Park et al. integrated tactile sensors into a Ferris wheel-shaped island elastic substrate, creating an e-skin capable of detecting tactile stimuli (Fig. 7(a) to 7(d)) [18]. Pressure sensors with micro-pyramid structures were strategically placed on the island structure to ensure their operation regardless of strain. Tensile sensors based on a porous carbon nanotube-Ecoflex composite material were coated on an Ecoflex substrate to detect strain. The electronic skin was attached to a human wrist, and pulse signals were successfully detected. Furthermore, it accurately distinguished various tactile stimuli applied to the e-skin as pressure and tensile signals, demonstrating its potential for use in next-generation stretchable electronics. Seo et al. developed a high-performance e-skin using dynamically cross-linked polyborosiloxane (PBS) and hybrid-polymerized Ecoflex composites [28]. The polymerization of PBS and Ecoflex was structurally designed through a double networking of dynamically cross-linked and covalently cross-linked networks. This e-skin exhibited rapid self-healing capability (within 30 s at room temperature), high stretchability (over 500%), high strength (maximum tensile stress of approximately 80 KPa), transparency (90% visible light transmittance), and excellent thermal stability (over 100 °C). The team utilized pressure sensors composed of this composite to create an e-skin capable of detecting body movements (Fig. 7(e) to 7(g)).
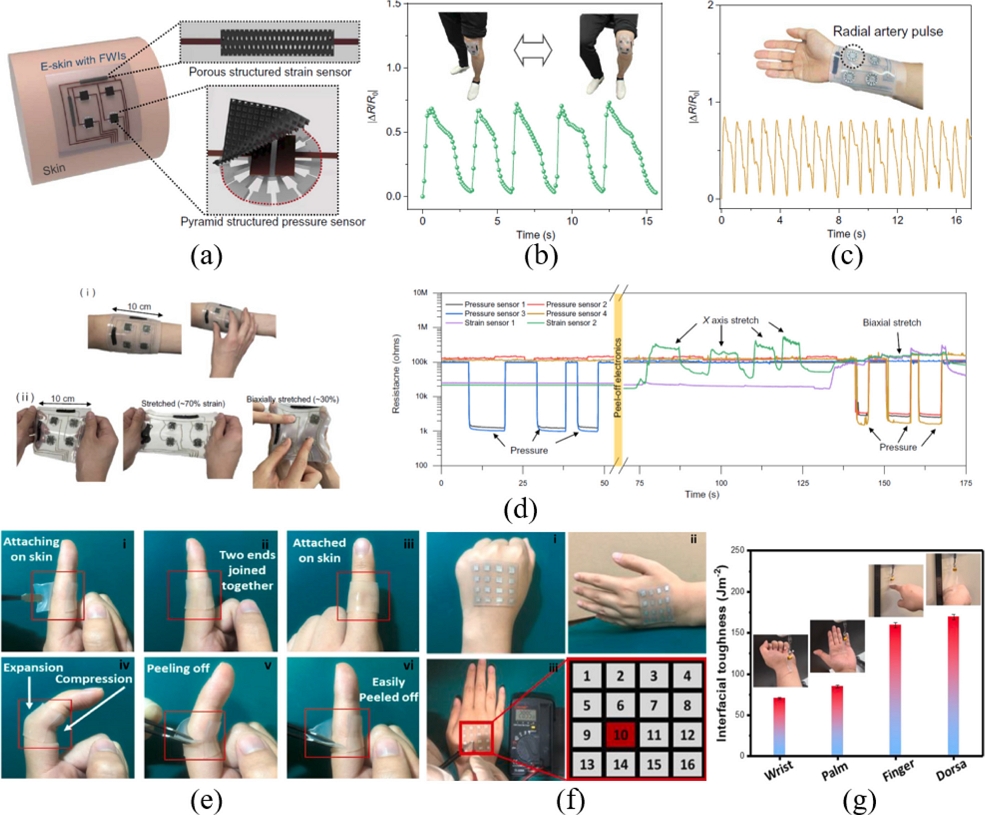
Health monitoring of e-skin. (a) Schematic of the pressure and strain sensors [18]. (b) Detection of a signal resulting from knee bending using strain sensor. (c) Detection of the radial artery pulse signal using a pressure sensor. (d) Real-time monitoring of pressure and lateral strain during various deformations. (e) Demonstration of the self-adhesive property and durability of our developed substrate: The substrate adheres tightly to the skin when worn on the finger joint, even during finger movement (expansion-compression). Reprinted with permission from Ref. [28]. Copyright (2023) Elsevier Ltd. (f) Photographs of a 4 × 4 electrode array patterned on a substrate illustrating skin attachability. The inset schematic shows 16 pixels of the electrode array, with the highlighted pixels indicating the measurement location. (g) Assessment of interfacial toughness between the PBS:Ecoflex substrate and human skin.
4. CONCLUSIONS
In this paper, we reviewed representative geometric strategies for implementing stretchable electronics. The research emphasis should prioritize investigating methods to enhance the efficacy of stretchable materials using geometric design. Additionally, exploring the integration of flexible materials with geometric designs is crucial to enhance the properties of stretchable electronic components. The traditional materials used in stretchable electronics are often limited by their low elasticity, making their application in electronic components challenging. To overcome this limitation, the geometric strategies presented in this paper encompass various approaches for imparting elasticity to rigid materials. We summarize (1) the serpentine electrode structure, which designs rigid materials into snake-like shapes; (2) the kirigami electrode structure, which patterns (cuts) flexible substrates; and (3) the island structure strategy, which places existing functional chips on non-stretchable rigid substrates. Finally, we discussed the outstanding achievements of sophisticated stretchable hybrid electronic devices on platforms such as stretchable skin, displays, battery packs, and tactile sensors.
By integrating stretchable electronic component technology and electronic parts, we can implement more reliable flexible sensors and potentially achieve enhanced sensitivity for accurate biological signal acquisition from the human skin. This technology will enable the development of diverse displays in the future with various form factors. Ensuring high durability against repetitive and excessive mechanical deformation while distinguishing physical stimuli without signal interference, which is crucial for the practical utility of e-skin in various applications, such as human-machine interfaces, health monitoring, and robotics.
Acknowledgments
Author Contributions
S.L and K.P were involved in experiments, analysis, and discussion. S.L. and K.P contributed equally to this work.
Funding
This research was supported by the National Research Foundation of Korea (Grant Number: NRF-2022R1A2C2006076) and RS-2023-00258591.
Declarations of competing interests
The authors declare no conflict of interests.
REFERENCES
-
N. Matsuhisa, X. Chen, Z. Bao, and T. Someya, “Materials and structural designs of stretchable conductors”, Chem. Soc. Rev., Vol. 48, No. 11, pp. 2946-2966, 2019.
[https://doi.org/10.1039/C8CS00814K]
-
W. Wu, “Stretchable electronics: functional materials, fabrication strategies and applications”, Sci. Technol. Adv. Mater., Vol. 20, No. 1, pp. 187-224, 2019.
[https://doi.org/10.1080/14686996.2018.1549460]
-
R.-H. Kim, D.-H. Kim, J. Xiao, S.-I. Park, B. Panilaitis, R. Ghaffari, M. Li, B. H. Kim, Z. Liu, D. L. Kaplan, F. G. Omenetto, Y. Huang, Z. Kang, and J. A. Rogers, “Waterproof AlInGaP optoelectronics on stretchable substrates with applications in biomedicine and robotics”, Nat. Mater., Vol. 9, No. 11, pp. 929-937, 2010.
[https://doi.org/10.1038/nmat2879]
-
Y. Zhang, H. Fu, Y. Su, S. Xu, H. Cheng, J. A. Fan, K.-C. Hwang, J. A. Rogers, and Y. Huang, “Mechanics of ultra-stretchable self-similar serpentine interconnects”, Acta Mater., Vol. 61, No. 20, pp. 7816-7827, 2013.
[https://doi.org/10.1016/j.actamat.2013.09.020]
-
K.-I. Jang, S. Y. Han, S. Xu, K. E. Mathewson, Y. Zhang, J.-W. Jeong, G.-T. Kim, R. C. Webb, J. W. Lee, T. J. Dawidczyk, R. H. Kim, Y. M. Song, W.-H. Yeo, S. Kim, H. Cheng, S. I. Rhee, J. Chung, B. Kim, H. U. Chung, D. Lee, Y. Yang, M. Cho, J. G. Gaspar, R. Carbonari, M. Fabiani, G. Gratton, Y. Huang, and J. A. Rogers, “Rugged and breathable forms of stretchable electronics with adherent composite substrates for transcutaneous monitoring”, Nat. Commun., Vol. 5, No. 1, pp. 4779(1)-4779(10), 2014.
[https://doi.org/10.1038/ncomms5779]
-
D. H. Kim, J. Xiao, J. Song, Y. Huang, and J. A. Rogers, “Stretchable, curvilinear electronics based on inorganic materials”, Adv. Mater., Vol. 22, No. 19, pp. 2108-2124, 2010.
[https://doi.org/10.1002/adma.200902927]
-
J. A. Fan, W.-H. Yeo, Y. Su, Y. Hattori, W. Lee, S.-Y. Jung, Y. Zhang, Z. Liu, H. Cheng, L. Falgout, M. Bajema, T. Coleman, D. Gregoire, R. J. Larsen, Y. Huang, and J. A. Rogers, “Fractal design concepts for stretchable electronics”, Nat. Commun., Vol. 5, pp. 3266(1)-3266(8), 2014.
[https://doi.org/10.1038/ncomms4266]
-
R. Xu, A. Zverev, A. Hung, C. Shen, L. Irie, G. Ding, M. Whitmeyer, L. Ren, B. Griffin, J. Melcher, L. Zheng, X. Zang, M. Sanghadasa, and L. Lin, “Kirigami-inspired, highly stretchable micro-supercapacitor patches fabricated by laser conversion and cutting”, Microsyst. Nanoeng., Vol. 4, No. 1, pp. 36(1)-36(10), 2018.
[https://doi.org/10.1038/s41378-018-0036-z]
-
N. Qaiser, A. N. Damdam, S. M. Khan, S. Bunaiyan, and M. M. Hussain, “Design Criteria for Horseshoe and Spiral‐Based Interconnects for Highly Stretchable Electronic Devices”, Adv. Funct. Mater., Vol. 31, No. 7, p. 2007445, 2020.
[https://doi.org/10.1002/adfm.202007445]
-
T. Kim, H. Lee, W. Jo, T. S, Kim, and S. Yoo, “Realizing Stretchable OLEDs: A Hybrid Platform Based on Rigid Island Arrays on a Stress‐Relieving Bilayer Structure”, Adv. Mater. Technol., Vol. 5, No. 11, p. 2000494, 2020.
[https://doi.org/10.1002/admt.202000494]
-
B. Lee, H. Cho, S. Jeong, J. Yoon, D. Jang, D. K. Lee, D. Kim, S. Chung, and Y. Hong, “Stretchable hybrid electronics: combining rigid electronic devices with stretchable interconnects into high-performance on-skin electronics”, J. Inf. Disp., Vol. 23, No. 3, pp. 163-184, 2022.
[https://doi.org/10.1080/15980316.2022.2070291]
-
S. Y. Hong, J. Yoon, S. W. Jin, Y. Lim, S. J. Lee, G. Zi, and J. S. Ha, “High-density, stretchable, all-solid-state microsupercapacitor arrays”, ACS Nano, Vol. 8, No. 9, pp. 8844-8855, 2014.
[https://doi.org/10.1021/nn503799j]
-
H. Song, G. Luo, Z. Ji, R. Bo, Z. Xue, D. Yan, F. Zhang, K. Bai, J. Liu, X. Cheng, W. Pang, Z. Shen, and Y. Zhang, “Highly-integrated, miniaturized, stretchable electronic systems based on stacked multilayer network materials”, Sci. Adv., Vol. 8, No. 11, pp. eabm3785(1)-eabm3785(13), 2022.
[https://doi.org/10.1126/sciadv.abm3785]
-
X. Li, P. Zhu, S. Zhang, X. Wang, X. Luo, Z. Leng, H. Zhou, Z. Pan, and Y. Mao, “Self-Supporting, Conductor-Exposing, Stretchable, Ultrathin, and Recyclable Kirigami-Structured Liquid Metal Paper for Multifunctional E-Skin”, ACS Nano, Vol. 16, No. 4, pp. 5909-5919, 2022.
[https://doi.org/10.1021/acsnano.1c11096]
-
L. Yang, G. Zheng, Y. Cao, C. Meng, Y. Li, H. Ji, X. Chen, G. Niu, J. Yan, Y. Xue, and H. Cheng, “Moisture-resistant, stretchable NO(x) gas sensors based on laser-induced graphene for environmental monitoring and breath analysis”, Microsyst. Nanoeng., Vol. 8, No. 1, pp. 78(1)-78(12), 2022.
[https://doi.org/10.1038/s41378-022-00414-x]
-
B. Yang, Y. Zhao, M. U. Ali, J. Ji, H. Yan, C. Zhao, Y. Cai, C. Zhang, and H. Meng, “Asymmetrically Enhanced Coplanar-Electrode Electroluminescence for Information Encryption and Ultrahighly Stretchable Displays”, Adv. Mater., Vol. 34, No. 31, p. 2201342, 2022.
[https://doi.org/10.1002/adma.202201342]
-
A. Amiri, K. Bashandeh, R. Sellers, L. Vaught, M. Naraghi, and A. A. Polycarpou, “Fully integrated design of a stretchable kirigami-inspired micro-sized zinc–sulfur battery”, J. Mater. Chem. A, Vol. 11, No. 20, pp. 10788-10797, 2023.
[https://doi.org/10.1039/D2TA08544E]
-
J. C. Yang, S. Lee, B. S. Ma, J. Kim, M. Song, S. Y. Kim, D. W. Kim, T. S. Kim, and S. Park, “Geometrically engineered rigid island array for stretchable electronics capable of withstanding various deformation modes”, Sci. Adv., Vol. 8, No. 22, pp. eabn3863(1)-eabn3863(11), 2022.
[https://doi.org/10.1126/sciadv.abn3863]
-
Y. Zhang, S. Xu, H. Fu, J. Lee, J. Su, K. C. Hwang, J. A. Rogers, and Y. Huang, “Buckling in serpentine microstructures and applications in elastomer-supported ultra-stretchable electronics with high areal coverage”, Soft Matter, Vol. 9, No. 33, pp. 8062-8070, 2013.
[https://doi.org/10.1039/c3sm51360b]
-
S. M. Curtis, J. L. Gugat, L. Bumke, D. Dengiz, L. Seigner, D. Schmadel, N. S. Lazarus, and E. Quandt, “Thin-Film Superelastic Alloys for Stretchable Electronics”, Shape Mem. Superelasticity, Vol. 9, No. 1, pp. 35-49, 2023.
[https://doi.org/10.1007/s40830-023-00422-4]
-
J. H. Song, Y. G. Kim, Y. Cho, S. Hong, J. Y. Choi, M. S. Kim, and S. H. Ahn, “Stretchable Strain and Strain Rate Sensor Using Kirigami‐Cut PVDF Film”, Adv. Mater. Technol., Vol. 8, No. 6, p. 2201112, 2023.
[https://doi.org/10.1002/admt.202201112]
-
K. B. Kim, Y. J. Lee, A. Costa, Y. K. Lee, T. S. Jang, M. G. Lee, Y. C. Joo, K. H. Oh, J. Song, and I. S. Choi, “Extremely Versatile Deformability beyond Materiality: A New Material Platform through Simple Cutting for Rugged Batteries”, Adv. Eng. Mater., Vol. 21, No. 7, p. 1900206(1)-1900206(8), 2019.
[https://doi.org/10.1002/adem.201900206]
-
K. Li, Y. Shuai, X. Cheng, H. Luan, S. Liu, C. Yang, Z. Xue, Y. Huang, and Y. Zhang, “Island Effect in Stretchable Inorganic Electronics”, Small, Vol. 18, No. 17, p. 2107879, 2022.
[https://doi.org/10.1002/smll.202107879]
-
X. Song, T. Zhang, L. Wu, R. Hu, W. Qian, Z. Liu, J. Wang, Y. Shi, J. Xu, K. Chen, and L Yu, “Highly Stretchable High-Performance Silicon Nanowire Field Effect Transistors Integrated on Elastomer Substrates”, Adv. Sci., Vol 9, No. 9, pp. 2105623(1)-2105623(9), 2022.
[https://doi.org/10.1002/advs.202105623]
-
S. Biswas, A. Schoeberl, Y. Hao, J. Reiprich, T. Stauden, J. Pezoldt, and H. O. Jacobs, “Integrated multilayer stretchable printed circuit boards paving the way for deformable active matrix”, Nat. Commun., Vol. 10, No. 1, pp. 4909(1)-4909(8), 2019.
[https://doi.org/10.1038/s41467-019-12870-7]
-
X. Ma, X. Wu, S. Cao, Y. Zhao, Y. Lin, Y. Xu, X. Ning, and D. Kong, “Stretchable and Skin-Attachable Electronic Device for Remotely Controlled Wearable Cancer Therapy”, Adv. Sci., Vol. 10, No. 10, pp. 2205343(1)-2205343(9), 2023.
[https://doi.org/10.1002/advs.202205343]
-
Z. Li, A. Islam, M. D. Luigi, Y. Huang, and S. Ren, “Stretchable copper-nanocellulose paper heater”, Appl. Mater. Today, Vol. 31, p. 101740, 2023.
[https://doi.org/10.1016/j.apmt.2023.101740]
-
R. Yeasmin, S.-I. Han, L. T. Duy, B. Ahn, and H. Seo, “A Skin-like Self-healing and stretchable substrate for wearable electronics”, J. Chem. Eng., Vol. 455, p. 140543, 2023.
[https://doi.org/10.1016/j.cej.2022.140543]