
Electrochemical Corrosion Properties of Plasma Electrolytic Oxidation Coatings on AZ31 Mg Alloy in Sodium Silicate-based Electrolyte Containing NaAlO2
This is an Open Access article distributed under the terms of the Creative Commons Attribution Non-Commercial License(https://creativecommons.org/licenses/by-nc/3.0/) which permits unrestricted non-commercial use, distribution, and reproduction in any medium, provided the original work is properly cited.
Abstract
Plasma electrolytic oxidation (PEO) of AZ31B Mg alloys was performed in NaAlO2 additive-containing Na2SiO3-based electrolytes, and the electrochemical corrosion properties of the PEO coatings were investigated. The concentration of NaAlO2 additive was varied from 2 to 6 g/L. As the NaAlO2 additive concentration increased, the Al content increased, whereas the Si content decreased in the PEO coatings. The surface porosity of the PEO coatings was minimized at a NaAlO2 content of 4 g/L. Potentiodynamic and electrochemical impedance spectroscopy tests in Ringer’s solution indicated that the corrosion resistance of the AZ31B Mg alloy was significantly improved by the addition of 4 g/L NaAlO2 to Na2SiO3-based electrolytes.
Keywords:
NaAlO2 additive, Plasma electrolytic oxidation, AZ31B magnesium alloy, Corrosion resistance1. INTRODUCTION
Magnesium (Mg) is known for its high specific strength, low density, and high thermal conductivity [1]. In addition, Mg alloys have attracted considerable attention as lightweight metallic materials owing to their excellent strength-to-mass ratios, abundant resources, and recyclability [2-4]. These alloys can be applied in various fields, including automotive, aerospace, electronics, biomedical engineering, and construction industries [3-6]. In addition, because of their low corrosion resistance [4], Mg alloys are candidates for concrete-corrosive sensor materials and are susceptible to chlorides [7]. In general, surface modification of Mg alloys can improve their anticorrosive performance, resulting in an extended lifespan under natural exposure conditions.
Among the number of surface modification processes, plasma electrolytic oxidation (PEO) is a cost-effective and environmentally friendly electrochemical process that modifies the surfaces of metal alloys, including Mg alloys, using ceramic-like coatings on their surfaces to improve corrosion and wear resistance [4,8-12]. The characteristics of PEO coatings are strongly influenced by the electrolyte [13-16]. The properties of the PEO coating can be improved by adding additives to alkaline electrolytes, such as silicate and phosphate systems. G. H. Lv et al. [17] found that the addition of 8 g/L sodium aluminate (NaAlO2) to a phosphate-based electrolyte improved the corrosion resistance of PEO coatings on the AZ91D magnesium alloy. In addition, Veys-Renaux et al. [18] reported the effect of 0.2 M NaAlO2 in a phosphate-based electrolyte on the corrosion behavior of the AZ91 Mg alloy. Although the sodium aluminate additive was not associated with the best anticorrosion performance, the presence of NaAlO2 as an additive in the electrolyte enhanced the corrosion resistance of the PEO coatings. Despite some publications devoted to the effect of sodium aluminate additives, it is often difficult to understand the influence of the NaAlO2 concentration on the characteristics of PEO coatings.
In this study, oxide coating was performed using the PEO method on an AZ31B Mg alloy in a sodium silicate-based electrolyte containing NaAlO2 as an additive, and the influence of the NaAlO2 concentration on the corrosion characteristics of the Mg alloy was investigated. The corrosion resistance properties were evaluated by potentiodynamic tests and electrochemical impedance spectroscopy (EIS). The PEO-coated Mg alloys have the potential to be used in the packaging or as a reference sensing material in the Mg-based corrosion sensor.
2. EXPERIMENTAL
AZ31B Mg alloy (Al 2.5-3.5 wt%, Zn 0.6-1.4 wt%, Mn 0.2-1.0 wt%, and Mg balance) substrates with sizes of 25 × 25 × 1 mm3 were used as working electrodes. The substrates were progressively polished with SiC abrasive papers up to 1500 grids. To remove surface contamination before the PEO coating, the sample substrates were ultrasonically cleaned with acetone and rinsed with distilled water. The sodium silicate-based aqueous solution with the composition of 1 g/L sodium hydroxide (NaOH) and 12 g/L sodium metasilicate nonahydrate (Na2SiO3·9H2O) was used as the electrolyte. Different concentrations of sodium aluminate in the range of 2–6 g/L were used as additives in the base electrolyte. A stainless-steel bath was used as the counter electrode. The distance between the working electrode and the counter was 45 mm. The PEO was performed for 30 s at a constant DC voltage of 240 V. Substrates with PEO-coated surfaces were rinsed with water and air-dried.
A field mission scanning electron microscope (FE-SEM, Hitachi S4200) with an energy-dispersive X-ray spectrometer (EDS, Horiba Inc.) was used for surface analysis. The surface and cross-sectional morphologies of the PEO coatings were investigated using FE-SEM. Surface porosity was analyzed from the surface morphologies using image analysis software. The PEO coating thickness was measured from the cross-sectional FE-SEM micrographs. EDS was performed to investigate the elemental composition of the surfaces of the PEO coatings.
The corrosion resistance was analyzed based on the electrochemical response of the PEO coatings using potentiodynamic polarization tests and EIS in Ringer's solution [19]. The electrochemical measurements were performed using a potentiostat equipped with a three-electrode cell system. A PEO layer with an exposed area of 1 cm2 was used as the working electrode and a platinum plate was used as the counter electrode. Ag/AgCl was used as the reference electrode. All the measurements were performed at room temperature.
3. RESULTS AND DISCUSSIONS
Fig. 1 shows the typical EDS spectra of the PEO coatings on the AZ31B Mg alloy. All the coatings contained four elements: Mg, O, Al, and Si. In the PEO coating with the base electrolyte, Mg and Al originate from the alloy substrate, whereas O and Si originate from the electrolyte. It can be seen that the peak intensity of Al is lower than that of Si, as shown in Fig. 1 (a). For the PEO coating with 6 g/L NaAlO2 as an additive, as shown in Fig. 1 (b), the peak intensity of Al was higher than that of Si because of the incorporation of Al ions or molecules from the electrolyte into the PEO coating. The elemental compositions of the PEO coatings with different concentrations of sodium aluminate are summarized in Table 1. As the sodium aluminate concentration increased, the aluminum content increased, whereas the silicon content decreased. However, there were no significant changes in the magnesium and oxygen contents.

EDS analysis of the PEO coatings with various concentrations of sodium aluminate and without sodium aluminate.
The morphologies of the PEO-coated surfaces with different concentrations of sodium aluminate in the range of 2–6 g/L were investigated using FE-SEM (Fig. 2). As can be seen, the PEO coatings with NaAlO2 additive significantly reduced the numbers and size of micropores, compared to the PEO coating of without sodium aluminate. The porosities of the PEO coatings with sodium aluminate concentrations of 0, 2, 3, 4, 5, and 6 g/L are 3.726%, 2.684%, 2.585%, 1.346%, 1.616%, and 3.046%, respectively. As the sodium aluminate concentration in the electrolyte increases from 0 to 4 g/L, the porosity decreases. This is because the increase in the sodium aluminate content caused intensive discharging sparks, increasing the electrical conductivity of the electrolytes [20]. In addition, the increased conductivity promotes denser and smaller pore formation owing to the addition of sodium aluminate, as shown in Fig. 2 [3]. However, the pore sizes of the PEO coatings increased at 5 and 6 g/L. This is also caused by excessive conductivity resulting from increased sparks [4]. Among the various concentrations of sodium aluminate, the PEO coating with a sodium aluminate concentration of 4 g/L exhibited the lowest porosity and the best uniformity.
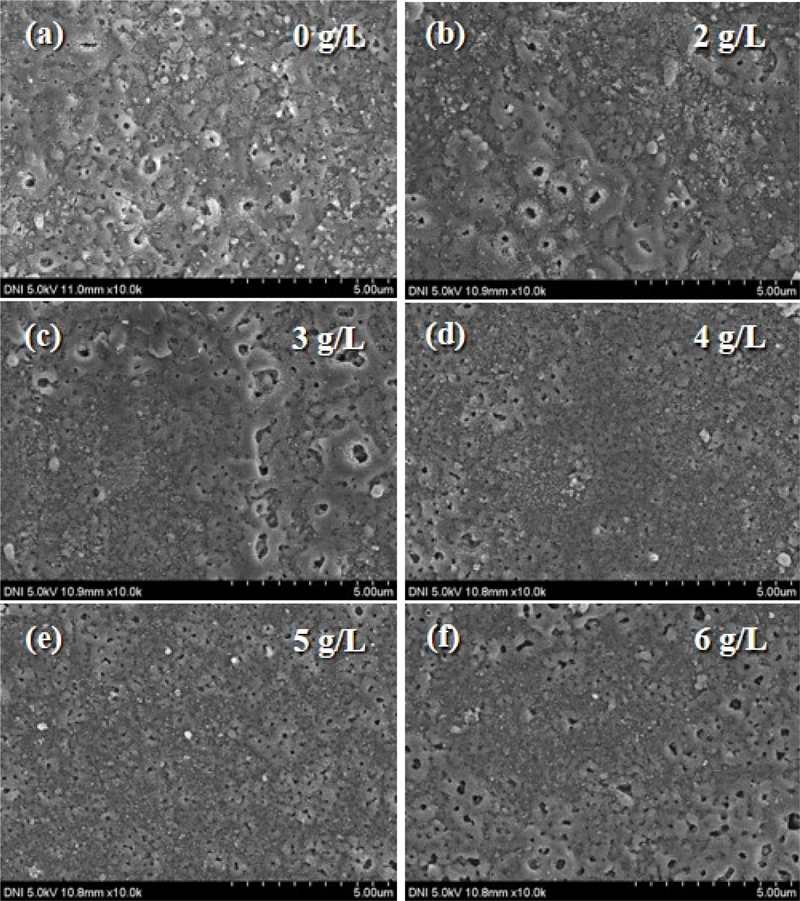
FE-SEM images of PEO-coated surfaces with different concentrations of sodium aluminate in the range of 2–6 g/L and without sodium aluminate.
Fig. 3 shows the potentiodynamic polarization curves of the PEO coatings in the Ringer’s solution. With an increase in the additive concentration to 4 g/L, the polarization curves exhibited a lower current density and higher potential, indicating that the corrosion resistance was significantly improved. At an additive content of 5 g/L, the current density started to increase and the potential started to decrease. The parameters related to corrosion characteristics such as corrosion potential (Ecorr), corrosion current density (Icorr), and anodic/cathodic Tafel constant (βa and βc) were derived from these data by Tafel extrapolation method, and the data are listed in Table 2. Polarization resistance (Rp) was calculated using the Stern-Geary equation [21]. For a sodium aluminate concentration of 4 g/L, Ecorr was -1.3215 V, which was higher than that of the other specimens. This results from the fact that the lower porosity minimized the Mg dissolution. For a sodium aluminate concentration of 4 g/L, Icorr was 4.378 ×10-8 A/cm2 which is lower than that of the other specimens. This implies that the PEO-coated layer could insulate the Mg alloy. Additively, the Rp is 2.875 ×105 Ω which is higher than that of the other specimens, which tells the PEO-coated layer with a concentration of 4 g/L sodium aluminate has better corrosion resistance among the specimens [9].
A Nyquist diagram of the EIS results for the PEO coatings with various sodium aluminate concentrations is shown in Fig. 4. In the high-frequency region, the Nyquist plots show depressed capacitive semicircles. The size of the radius is closely related to the corrosion resistance. As the resistance increases, the radius also increases; thus, the larger the radius, the better the corrosion resistance [9]. As shown in Fig. 4, the radii of the PEO coating with the sodium aluminate additive are larger than those of the PEO coating without sodium aluminate in the base electrolyte, indicating an improvement in the corrosion resistance. Among the PEO-coated layers with various concentrations of sodium aluminate, the PEO-coated layer with 4 g/L sodium aluminate exhibited the largest radius, implying that its corrosion resistance was the best among these specimens.
4. CONCLUSIONS
The effects of the sodium aluminate concentration in NaOH-Na2SiO3-NaAlO2 electrolytes on the electrochemical corrosion properties of PEO coatings on AZ31B Mg alloys were investigated. The EDS spectra explain that adding NaAlO2 into the silicate-based electrolytes increased the Al content of the PEO coating. The morphologies of the PEO-coated surfaces with different concentrations of sodium aluminate were investigated using FE-SEM. Up to a NaAlO2 content of 4 g/L, the porosity of the PEO surface decreased. At 4 g/L of NaAlO2 concentration in the electrolyte, the images show a relatively smaller pore size and porosity of the PEO coating. From the potentiodynamic polarization curves, Ecorr was the highest and Icorr was the lowest at 4 g/L of NaAlO2 concentration in the electrolyte among the specimens. Among the PEO-coated layers with various concentrations, the PEO-coated layer with 4 g/L sodium aluminate exhibited the largest radius in Nyquist plots. This electrical analysis implies that the corrosion resistance was the best among the samples. At 4 g/L of NaAlO2 concentration in the electrolyte, the highest corrosion resistance of the PEO coating was obtained at a NaAlO2 concentration of 4 g/L in the electrolyte. Therefore, it is concluded that adding NaAlO2 with proper content in the silicate-based electrolytes can lead to an increase in the corrosion resistance of AZ31B Mg alloy coatings. This PEO-coated layer could be applied in packaging and as a reference sensing element for Mg-based corrosion sensors.
Acknowledgments
This work was supported by research grants from Daegu Catholic University in 2022.
REFERENCES
-
Y. He, L. Wu, W. Yao, Y. Yuan, R. Wu, G. Wu, J. Wang, and F. Pan, “Analysis of the evolution process of corrosion products of pure magnesium based on the phase angle”, Corros. Sci., Vol. 240, pp. 112492(1)-112492(10), 2024.
[https://doi.org/10.1016/j.corsci.2024.112492]
-
A. Atrens, G. L. Song, M. Liu, Z. M. Shi, F. Y. Cao, and M. S. Dargusch, “Review of recent developments in the field of magnesium corrosion”, Adv. Eng. Mater., Vol. 17, pp. 400-453, 2015.
[https://doi.org/10.1002/adem.201400434]
-
T. T. Thanaa, A. Fattah-alhosseini, M. Alkaseem, and M. Kaseem, “Improving the surface properties of Mg based-plasma electrolytic oxidation (PEO) coatings under the fluoride electrolytes: A review”, Inorg. Chem. Commun., Vol. 170, pp. 113163(1)-113163(18), 2024.
[https://doi.org/10.1016/j.inoche.2024.113163]
-
K. D. Moon, and D. K. Lee, “Effects of the sodium borate additions in NaOH-Na2SiO3 electrolytes on the surface and corrosion properties of plasma electrolytic oxidation coatings on AZ31Mg alloy”, Mol. Cryst. Liq. Cryst., Vol. 735, pp. 15421406(1)-15421406(7), 2021.
[https://doi.org/10.1080/15421406.2021.1972221]
-
C. Jiang, B. Lu, H. Pan, Y. Zhang, Z. Zeng, Q. Huang, W. Tang, N. Mo, Y. Xu, and G. Qin, “Effect of Al addition on microstructure and mechanical property of Mg-Mn-Ca-Ce based alloy”, J. Alloys Compd., Vol. 1005, pp. 175975(1)-175975(8), 2024.
[https://doi.org/10.1016/j.jallcom.2024.175975]
-
S. F. Hassan, M. T. Islam, S. Nouari, M. M. A. Baig, and F. Patel, “The role of calcium addition through powder sintering process in microstructure and mechanical behavior of a microalloyed magnesium,” Mater. Today Commun., Vol. 41, pp. 110312(1)-110312(11), 2024.
[https://doi.org/10.1016/j.mtcomm.2024.110312]
-
L. Yan, G.-L. Song, P. Wu, Y. Zhu, and D. Zheng, “A chloride-sensitive corrosion sensor and protector made of an optimized Mg-Al alloy for reinforcing steel”, Mater. Des., Vol. 210, pp. 110028(1)-110028(16), 2021.
[https://doi.org/10.1016/j.matdes.2021.110028]
-
D. K. Lee, D. R. Hwang, and Y.-S. Sohn, “Surface properties of plasma electrolytic oxidation coatings on Ti-alloy in phosphate–based electrolytes with the addition of sodium metasilicate”, Mol. Cryst. Liq. Cryst., Vol. 687, No. 1, pp. 7-13, 2019.
[https://doi.org/10.1080/15421406.2019.1648048]
-
M. Daroonparvar, M. A. M. Yajid, N. M. Yusof, and H. R. Bakhsheshi-Rad, “Preparation and corrosion resistance of a nanocomposite plasma electrolytic oxidation coating on Mg-1%Ca alloy formed in aluminate electrolyte containing titania nano-additives”, J. Alloys Compd., Vol. 688, pp. 841-857, 2016.
[https://doi.org/10.1016/j.jallcom.2016.07.081]
-
R. O. Hussein, D. O. Northwood, and X. Nie, “The effect of processing parameters and substrate composition on the corrosion resistance of plasma electrolytic oxidation (PEO) coated magnesium alloys”, Surf. Coat. Technol., Vol. 237, pp. 357-368, 2013.
[https://doi.org/10.1016/j.surfcoat.2013.09.021]
-
P. Zhang, X. Nie, and D. O. Northwood, “Influence of coating thickness on the galvanic corrosion properties of Mg oxide in an engine coolant”, Surf. Coat. Technol., Vol. 203, pp. 3271-3277, 2009.
[https://doi.org/10.1016/j.surfcoat.2009.04.012]
-
M. Kaseem, K. Choi, and Y. G. Ko, “A highly compact coating responsible for enhancing corrosion properties of Al-Mg-Si alloy”, Mater. Lett., Vol. 196, pp. 316-319, 2017.
[https://doi.org/10.1016/j.matlet.2017.03.106]
-
S. Aliasghari, R. Skeldon, and G. E. Thompson, “Plasma electrolytic oxidation of titanium in a phosphate/silicate electrolyte and tribological performance of the coatings”, App. Surf. Sci., Vol. 316, pp. 463-476, 2014.
[https://doi.org/10.1016/j.apsusc.2014.08.037]
-
X. Yang, M. Li, X. Lin, L. Tan, G. Lan, L. Li, Q. Yin, H. Xia, Y. Zhang, and K. Yang, “Enhanced in vitro biocompatibility/bioactivity of biodegradable Mg–Zn–Zr alloy by micro-arc oxidation coating contained Mg2SiO4”, Surf. Coat. Technol., Vol. 233 pp. 65-73, 2013.
[https://doi.org/10.1016/j.surfcoat.2013.01.052]
-
A. Ghasemi, V. S. Raja, C. Blawert, W. Dietzel, and K. U. Kainer, “The role of anions in the formation and corrosion resistance of the plasma electrolytic oxidation coatings”, Surf. Coat. Technol., Vol. 204, pp. 1469-1478, 2010.
[https://doi.org/10.1016/j.surfcoat.2009.09.069]
-
S. V. Gnedenkov, O. A. Khrisanfova, A. G. Zavidnaya, S. L. Sinebryukhov, V. S. Egorkin, M. V. Nistratova, A. Yerokhin, and A. Matthews, “PEO coatings obtained on an Mg–Mn type alloy under unipolar and bipolar modes in silicate-containing electrolytes”, Surf. Coat. Technol., Vol. 204, pp. 2316-2322, 2010.
[https://doi.org/10.1016/j.surfcoat.2009.12.024]
-
G. H. Lv, H. Chen, X. Q. Wang, H. Pang, G. L. Zhang, B. Zou, H. J. Lee, and S. Z. Yang, “Effect of additives on structure and corrosion resistance of plasma electrolytic oxidation coatings on AZ91D magnesium alloy in phosphate based electrolyte”, Surf. Coat. Technol., Vol. 205, pp. S36-S40, 2010.
[https://doi.org/10.1016/j.surfcoat.2010.03.035]
-
D. Veys-Renaux, C.-E. Barchiche, and E. Rocca, “Corrosion behavior of AZ91 Mg alloy anodized by low-energy micro-arc oxidation: Effect of aluminates and silicates”, Surf. Coat. Technol., Vol. 251, pp. 232-238, 2014.
[https://doi.org/10.1016/j.surfcoat.2014.04.031]
-
M. Jamesh, S. Kumar, and T. S. N. Sankara Narayanan, “Corrosion behavior of commercially pure Mg and ZM21 Mg alloy in Ringer’s solution – Long term evaluation by EIS”, Corros. Sci., Vol. 53, pp. 645-654, 2011.
[https://doi.org/10.1016/j.corsci.2010.10.011]
-
R. H. U. Khan, A. Yerokhin, X. Li, H. Dong, and A. Matthews, “Surface characterisation of DC plasma electrolytic oxidation treated 6082 aluminium alloy: Effect of current density and electrolyte concentration”, Surf. Coat. Technol., Vol. 205, pp. 1679-1688, 2010.
[https://doi.org/10.1016/j.surfcoat.2010.04.052]
-
M. Stern and A. L. Geary, “Electrochemical polarization: I. A theoretical analysis of the shape of polarization curves”, J. Electrochem. Soc., Vol. 104, pp. 56-63, 1957.
[https://doi.org/10.1149/1.2428496]